The Carbohydrate Metabolism section provides High Yield information for the MCAT, Medical School, Residency, and in the future career as a Physician. Prepare and Learn Ahead! Educating, Preparing, and Proving high-yield content, quizzes, and medical resources. to students who are interested in the medical field.
Table Of Contents
Glucose Transport
- Glucose entry into most cells is driven by concentration and is independent of sodium
- Normal range is 4-6 mM
- There are four glucose transporters: GLUT 1 – GLUT 4
- GLUT 2 & 4 are the most significant since they are located only in specific cells and are highly regulated
- Glut 2: low-affinity transporter in hepatocytes and pancreatic cells
- Glut 2 captures excess glucose from the hepatic portal vein (coming from the intestine) and primarily stores it
- When glucose levels drop below the Km for the transporter, the remaining glucose will then bypass the liver and enter the peripheral circulation
- The KM for GLUT 2 is relatively high at approximately 15 mM
- Liver will pick up glucose in proportion to its concentration in blood
- Liver will pick up glucose and store it after a meal
- Glut 4: responds to the glucose concentration in peripheral blood
- Found in adipose tissue and muscles
- Rate of glucose transport to these two tissues is increased by insulin
- This then adds additional GLUT 4 transporters to the membrane
- KM of GLUT 4 is similar to normal glucose levels in blood
- Means that the transporter is saturated at blood glucose levels that are a bit higher than normal
- Thus at higher glucose levels, the transporters will still permit only a constant rate of glucose influx (zero-order kinetics)
- Cells with GLUT 4 transporters thus increase their intake of glucose by increasing the number of transporters on their surface (since the transporters are already saturated at normal glucose levels)
- Glucose transport occurs at basal levels at all times, but insulin has the ability to increase the transport rate in adipose tissue and muscles
- Muscles store excess glucose as glycogen and adipose tissue requires glucose to store fatty acids as triacylglycerols
Glycolysis
- A cytoplasmic pathway that converts glucose into two pyruvate molecules
- Releases a moderate amount of energy through two substrate-level phosphorylations and one oxidation reaction
- If a cell has mitochondria and oxygen, the product of glycolysis (NADH) can be fed into the aerobic respiration pathway in order to generate energy for the cell
- If no mitochondria or oxygen, then glycolysis can occur anaerobically, results in much smaller energy output
- Also a part of converting excess glucose into fatty acids
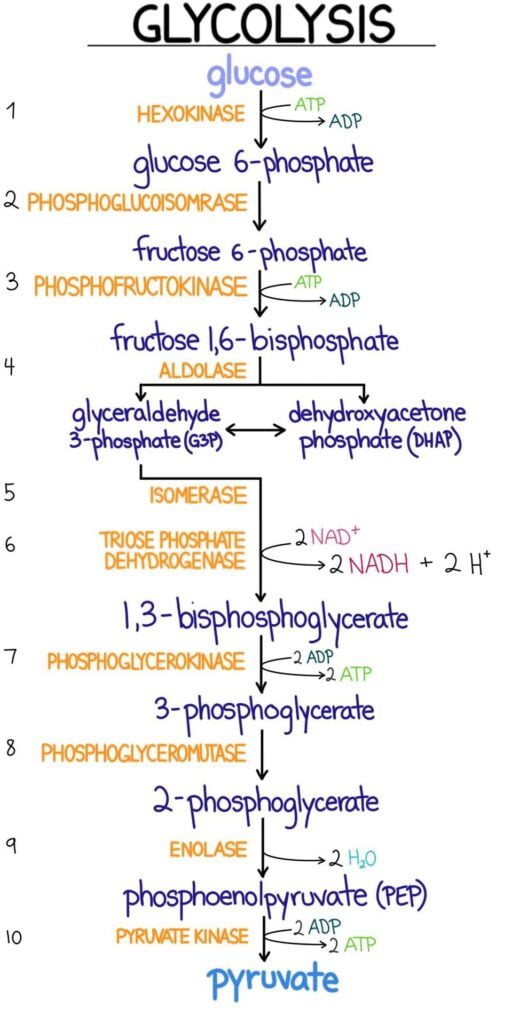
Important Enzymes of Glycolysis
Hexokinase and Glucokinase
- First step in glucose metabolism is to transport it across the membrane and then phosphorylate it by kinase enzymes
- Phosphorylation prevents glucose from leaving via a transporter
- Kinases attach a phosphate group from ATP to their substrates
- Glucose enters the cell by facilitated diffusion or active transport
- Kinases convert glucose to glucose 6-phosphate
- GLUT transporters are specific for glucose and not for phosphorylated glucose, so the converted glucose cannot leak out of the cell.
- Hexokinase: widely distributed and is inhibited by its product, glucose 6-phosphate
- Glucokinase: found in liver cells and pancreatic beta-islet cells
- In liver, kinase is induced by insulin
Phosphofructokinase (PFK-1 & PFK-2)
- Phosphofructokinase-1: Rate limited enzyme and main control point of glycolysis
- Reaction involves fructose 6-phosphate being phosphorylated to fructose 1,6-biphosphate by using ATP
- PFK-1 is inhibited by ATP and citrate, and activated by AMP
- Cell should turn off glycolysis when it has sufficient energy and turns on glycolysis when it needs energy (high AMP)
- Citrate is an intermediate of the citric cycle, implies that the cell is producing enough energy
- Insulin stimulates and glucagon inhibits PFK-1 in hepatocytes indirectly through PFK-2 and fructose 2,6-biphosphate
- Insulin activates PFK-2, which then converts a tiny amount of fructose 6-phosphate to fructose 2,6-biphosphate.
- F2,6-BP activates PFK-1
- Glucagon inhibits PFK-2, which lowers the levels of F2,6-BP which then inhibits PFK-1.
- PFK-2 is found mainly in the liver
- PFK-1 allows for cells to override the inhibition caused by ATP so that glycolysis can continue
Glyceraldehyde-3-Phosphate Dehydrogenase
- Catalyzes an oxidation and addition of an inorganic phosphate (Pi) to its substrate: glyceraldehyde 3-phosphate.
- Results in the production of a high energy intermediate: 1,3-biphosphoglycerate and also the reduction of NAD+ to NADH
- NADH can then be oxidized by mitochondrial electron transport chain, if oxygen is present.
3-Phosphoglycerate Kinase
- Transfers high-energy phosphate from 1,3-biophosphoglycerate to ADP
- This results in the formation of ATP and 3-phosphoglycerate
- This is an example of a substrate-level phosphorylation reaction: ADP is directly phosphorylated to ATP by using a high-energy intermediate
- This is not dependent on oxygen, so it is the only means of ATP generation in anaerobic tissue
Pyruvate Kinase
- Last enzyme in aerobic glycolysis
- Catalyzes a substrate-level phosphorylation of ADP using the high-energy substrate phosphoenolpyruvate (PEP)
- Pyruvate Kinase: activated by fructose 1,6-biphosphate from the PFK-1 reaction
- Feed-Forward activation: products of an earlier reaction of glycolysis stimulate, or prepare, a later reaction in glycolysis
Fermentation
- Fermentation occurs in the absence of oxygen
- Lactate dehydrogenase oxidizes NADH to NAD+, this replenishes the oxidized coenzyme for glyceraldehyde-3-phosphate dehydrogenase
- Without mitochondria/oxygen, glycolysis would stop when all NAD+ is converted to NADH
- Lactate dehydrogenase prevents this from occurring by reducing pyruvate to lactate and thus oxidizing NADH to NAD+
- Result of fermentation is always the same: to oxidize NADH
Important Intermediates of Glycolysis
- Dihydroxyacetone phosphate (DHAP): used in hepatic and adipose tissue for triacylglycerol synthesis.
- DHAP is formed from fructose 1,6-bisphosphate
- It can then be isomerized to glycerol 3-phosphate, which can then be converted to glycerol
- 1,3-Biphosphoglycerate (1,3-BPG) & Phosphoenolpyruvate (PEP): High energy intermediates used to generate ATP by substrate-level phosphorylation
- Only ATP is gained in anaerobic respiration.
Irreversible Enzymes
- Three enzymes catalyze reactions that are irreversible, which thus keeps the pathway moving in only one direction
- Different enzymes need to be used if the liver intends to under gluconeogenesis (opposite of glycolysis).
- Different reaction need to be conducted at three key steps of glycolysis: Glucokinase/hexokinase, PFK-1, Pyruvate kinase
- Above three enzymes catalyze an irreversible reaction
Glycolysis in Erythrocytes
- Anaerobic glycolysis yields a net 2 ATP per glucose
- RBC’s have biphosphoglycerate mutase, this produces 2,3-biphosphoglycerate (2,3-BPG) from 1,3-BPG in glycolysis
- Mutases are enzymes that move a functional group from one place in a molecule to another
- 2,3-BPG binds to the beta-chains of hemoglobin A and decreases its affinity for oxygen
- Causes a rightward shift in the oxygen dissociation curve for HbA
- Allows for the unloading of oxygen in tissues, but allows it to be 100 percent saturated in the lungs
- 2,3-BPG does not bind to fetal hemoglobin as well. This means that the HbF has a higher affinity for oxygen than the maternal HbA.
- It allows for the transplacental passage of oxygen from mother to fetus.
Other Monosaccharides
- Glucose is the main source of ATP production in the body, but other monosaccharides like galactose and fructose can also contribute by feeding into glycolysis or other metabolic processes.
Galactose Metabolism
- Lactase is hydrolyzed to galactose and glucose by lactase
- Galactose reaches the liver through the hepatic portal vein, and can be transported to a tissue
- Galactose is phosphorylated by galactokinase (traps it inside the cell)
- Results in galactose 1-phosphate
- Galactose-1-phosphate uridyltransferase and an epimerase convert galactose 1-phosphate to glucose 1-phosphate
- Emimerases: enzymes that catalyze the conversion of one sugar epimer to another
Fructose Metabolism
- Fructose is found in honey and fruits and as part of the disaccharide sucrose (sugar)
- Sucrose is hydrolyzed by the brush border enzyme sucrose and the resulting glucose and fructose is absorbed into the hepatic portal vein
- Fructokinase: phosphorylates fructose (traps it inside of the cell by turning it into fructose 1-phosphate)
- Aldolase B: cleaves the F1-P into glyceraldehyde and DHAP
Pyruvate Dehydrogenase
- In aerobic glycolysis, pyruvate enters mitochondria and is converted to acetyl-CoA, which can enter into the citric acid cycle (if ATP needed), or for fatty acid synthesis (if there is sufficient ATP)
- Pyruvate dehydrogenase complex (PDH): An irreversible reaction and cannot be used to convert acetyl-CoA to pyruvate or glucose
- Pyruvate dehydrogenase is activated by insulin in the liver and is not responsive to hormones in the nervous system
- High insulin levels signal that the individual is in a well-fed state
- Liver should burn glucose for energy
- Shift fatty acid equilibrium towards production and storage
- High insulin levels signal that the individual is in a well-fed state
- Pyruvate dehydrogenase is one of three possible fates for pyruvate, the other two are: pyruvate conversion to lactate by lactate dehydrogenase or conversion to oxaloacetate by pyruvate carboxylase
- Overall complex requires multiple cofactors and coenzymes
- E.g. – thiamine, pyrophosphate, lipoic acid, CoA, FAD, and NAD+
- Pyruvate dehydrogenase is inhibited by its product, Acetyl-CoA.
- Buildup of acetyl-CoA causes a shift in metabolism from producing acetyl-CoA (for citric acid cycle) to producing oxaloacetate (enters gluconeogenesis)
Glycogenesis and Glycogenolysis
- Glycogen: branched polymer of glucose and is its storage form.
- Synthesis and degradation occurs mainly in the liver and skeletal muscle
- Stored in the cytoplasm as granules
- Each granule has a central protein core with poly-glucose chains radiating outwards to form a sphere
- If entirely composed of linear chains, glucose is highest at the core
- If branched chains, glucose density is highest at the periphery
- Glycogen in the liver is a source of glucose that is mobilized between meals to prevent low blood sugar. Muscle glycogen is stored as an energy reserve for muscle contraction.
- Plants also store excess glucose in long linked chains: starch
Glycogenesis
- Synthesis of glycogen granules
- Begins with core protein: glycogenin
- Glucose addition to the granule begins with glucose 6-phosphate which is converted to glucose 1-phosphate
- G,1-P is then activated by coupling to a molecule of urine diphosphate (UDP)
- Permits the integration into the glycogen chain
- Activation occurs when G,1-P interacts with UTP which forms UDP-glucose and a pyrophosphate (PPi)
Glycogen Synthase
- Rate limiting enzyme of glycogen synthesis
- Forms the a-1,4 glycosidic bond that is found in the linear glucose chain of the granule
- Stimulated by glucose 6-phosphate and insulin
- Inhibited by epinephrine and glucagon
- Inhibited through a protein kinase cascade that phosphorylates and inactivates the enzyme
Branching Enzyme (Glycosyl a-1,4: a-1,6 Transferase)
- Responsible for introducing alpha-1,6-linked branches into the granule as it grows
- Hydrolyze one of the a-1,4 bonds to release a block of oligoglucose (glucose molecules bonded together in a chain), which is then moved and added in a slightly different location
- Forms an a-1,6 bond to create a branch
Glycogenolysis
- The process of breaking down glycogen
- Rate limiting enzyme is glycogen phosphorylase
- A phosphorylase breaks bonds using an inorganic phosphate
Glycogen Phosphorylase
- Breaks a-1,4 glycosidic bonds, which releases glucose 1-phosphate from the periphery of the granule.
- Unable to break a-1,6 bonds and must therefore stop as the outermost branch points
- Activated by glucagon in the liver, so that glucose can be provided to the rest of the body
- Activated by AMP & epinephrine in skeletal muscle: signals that the muscle is active and requires more glucose
- Inhibited by ATP
Debranching Enzyme
- Two enzyme complex that deconstructs the branches in glycogen:
- Break an a-1,4 bond adjacent to the branch point and moves the oligoglucose chain that is released to the exposed end of the other chain.
- Forms a new a-1,4 bond
- Hydrolyzes the a-1,6 bond. This releases the single glucose left at the branch point
- The only free glucose that is produced directly from Glycogenolysis
Glycogen Storage Diseases
- Clinical feature of metabolic glycogen defect depends on: which enzyme is affected, the degree to which that enzyme’s activity is decreased, and which isoform of the enzyme is affected.
- Isoforms: slightly different versions of the same protein
- Deficiencies are termed glycogen storage diseases since all are characterized by the accumulation or lack of glycogen in one or more tissues
Gluconeogenesis
- Liver maintains glucose levels in blood through either Glycogenolysis or gluconeogenesis (also happens to a lesser extent in the kidneys)
- Pathways are promoted by glucagon and epinephrine and inhibited by insulin
- During fasting, glycogen levels drop dramatically, after 24 hours, gluconeogenesis is the only source of glucose.
- Important substrates for gluconeogenesis are:
- Glycerol 3-phosphate (from stored fats or triacylglycerols in adipose tissue)
- Lactate
- Glucogenic amino acids (from muscle proteins)
- Glucogenic Amino Acids: can be converted into intermediates that feed into gluconeogenesis
- Includes all amino acids except for leucine and lysine
- Ketogenic Amino Acids: converted to ketone bodies, which can be used as an alternative fuel. Amino acids can be either of the above or both
- It is not possible to convert Acetyl-CoA back into glucose
- Most fatty acids metabolize into Acetyl-CoA, so they aren’t a glucose source either
- Only exception is for fatty acids with an odd number of carbon atoms. These yield propionyl-CoA (glucogenic)
- All of the important substrates are converted into glycolytic intermediates:
- Lactate is converted to pyruvate by lactate dehydrogenase
- Alanine is converted to pyruvate by alanine aminotransferase
- Glycerol 3-phosphate is converted to dihydroxyacetone phosphate (DHAP) by glycerol-3-phosphate dehydrogenase
Important Enzymes of Gluconeogenesis
- Gluconeogenesis is basically a reversal of glycolysis
- Four enzymes are used to circumvent the irreversible steps of glycolysis in the liver
Pyruvate Carboxylase
- Mitochondrial enzyme that is activated by acetyl-CoA
- Oxaloacetate (OAA) is the product. This is an intermediate of the citric acid cycle and it cannot leave the mitochondrion
- Can leave the mitochondrion by being reduced to malate
- Once in cytoplasm, malate is oxidized back to OAA
- Acetly-CoA inhibits pyruvate dehydrogenase since it implies that the cell is energetically satisfied. i.e. – tells the cell to stop burning glucose
- This allows pyruvate to help generate glucose through gluconeogenesis
- Acetyl-CoA is not from glycolysis/pyruvate dehydrogenase; it is instead from fatty acids being burned
- To produce glucose in the liver during gluconeogenesis, fatty acids must be burned to provide the energy, stop the forward flow of the citric acid cycle and produce massive amounts of OAA.
Phosphoenolpyruvate Carboxykinase (PEPCK)
- PEPCK is found in the cytoplasm and is induced by glucagon and cortisol
- Converts OAA to phosphoenolpyruvate (PEP) in a reaction that requires GTP
- PEP turns into fructose 1,6-biphosphate
- Combination of pyruvate carboxylase and PEPCK are used to circumvent the action of pyruvate kinase. Done by converting pyruvate back into PEP
Fructose 1,6-bisphosphatase
- Rate-limiting steps of gluconeogenesis when it is found in the cytoplasm
- Reverses the action of PFK-1 (rate-limiting step of glycolysis)
- Removes a phosphate form fructose 1,6-biphosphate to produce 6, phosphate
- Phosphatases oppose kinases
- Activated by ATP and inhibited by AMP/fructose 2,6 bisphosphate
- High level of ATP implies that the cell is satisfied and can make glucose for the rest of the body
- High level of AMP implies that cell needs energy and cannot afford to produce energy for the rest of the body
- High levels of F 2,6-BP stimulates PFK-1 which stimulates glycolysis
- Glucagon will lower F2,6-BP and stimulate gluconeogenesis
- Insulin will increase F2,6-BP and inhibit gluconeogenesis
Glucose-6-Phosphatase
- Found in the lumen of the ER in liver cells
- Glucose-6-phosphate is transported into the ER, and then free glucose is transported back into the cytoplasm, where it can then be diffused out using GLUT transporters
- Glucose-6-Phosphatase is used to circumvent glucokinase and hexokinase (convert glucose to glucose-6-phosphate)
- Alanine is the major glucogenic amino acid, but most of the other amino acids are also glucogenic. Most are converted to citric acid intermediates and then to malate
- Hepatic gluconeogenesis is always dependent on b-oxidation of fatty acids in the liver
- This is what provides the energy source for the liver to get glucose
- Acetyl-CoA form fatty acids can be converted into ketone bodies and be used as an alternative source of fuel
Pentose Phosphate Pathway
- The PPP is also known as the hexose monophosphate (HMP) shunt
- Occurs in the cytoplasm of all cells
- Serves two major functions:
- Production of NADPH
- Source of ribose 5-phosphate for nucleotide synthesis
- First part starts with glucose 6-phosphate and ends with ribulose 5-phosphate
- Irreversible and also produces NADPH
- Rate limiting enzyme is glucose-6-phosphate dehydrogenase (G6PD)
- G6PD is induced by insulin since an abundance of sugar entering a cell will cause it to be shunted into both fuel utilization and fuel storage pathways.
- Shunt is inhibited by its product, NADPH, but activated by its reactant: NADP+
- Second part of pathway is a set of reversible reactions that produces an equilibrated pool of sugars for biosynthesis.
- Ribose 5-phosphate is produced for nucleotide synthesis (backbone of nucleic acids)
- Fructose 6-phosphate and glyceraldehyde 3-phosphate are also produced, these intermediates can be fed back into glycolysis
- Pentoses can also be made up from the glycolytic intermediates
Functions of NADPH
- NAD+ acts as a high energy electron acceptor for biochemical reactions
- Thought of as a potent oxidizing agent
- NADH formed from reduction reaction can be fed into electron transport to indirectly produce ATP
- NADPH primarily acts as an electron donor in a number of biochemical reaction
- Potent reducing agent
- Required for a variety of reasons:
- Biosynthesis of fatty acids and cholesterols
- Cellular bleach production in certain W.B.C’s
- Maintenance of a supply of reduced glutathione: helps protect against reactive oxygen species.
- Protects cell from free radical damage caused by peroxides
- Free radicals can attack lipids (including those in the membrane), and thus reduce or eliminate their functionality
- Can damage DNA and potentially cause cancer
- Glutathione is a reducing agent that helps reverse radical formation